Our research team is based on Atomic and Molecular Physics Beamline Station at National Synchrotron Radiation Laboratory (NSRL), and our interests are centered on atmospheric and combustion chemistry. The atomic and molecular physics beamline provides a continuous, tunable, high photon flux vacuum ultraviolet (VUV) light with energy ranging from 7 to 124 eV. Upon recent upgrades, we have now formed three research directions: gas-phase chemical reaction kinetics, reaction regulation and energy conversion in combustion, component analysis of complex chemical systems. In addition, we have capacities to carry out research on atomic and molecular physics, cluster science.
Atomic and Molecular Physics Beamline:http://www.nsrl.ustc.edu.cn/10960/list.htm
Gas phase chemical reaction kinetics
Gas phase chemical reaction kinetics is a subject that studies the rate of chemical reaction and the influence of various factors on the rate of chemical reaction. For complex chemical reaction systems such as atmospheric chemistry and combustion chemistry, the reaction network consists of many elementary reactions and multiple intermediates. The core parts are rate constants and branching fractions. In order to construct these reaction networks and further develop reliable theoretical models for real atmospheric and combustion applications, we design different reactors to simulate the chemical reactions of interest (such as from free radicals, new fuels, etc.) at wide ranges of temperatures and pressures. In such way, the complicated atmosphere chemistry and combustion flame are simplified, which facilitate the measurements of the key components and parameters of the sub-reactions. Equipped with the synchrotron radiation VUV photoionization mass spectrometry (SR-VUV-PIMS), time-resolved laser spectroscopy and other comprehensive analytic methods, our group have strong capability to explore the complicated chemical reaction kinetic process in detail.
Projects: Atmospheric chemistry, Combustion chemistry, Theoretical calculation and chemical kinetics simulation
Atmospheric chemistry
Highly reactive free radicals can significantly affect the gas-phase chemical environment, such as the atmosphere, combustion, and interstellar media. In order to accurately simulate these systems, we need detailed chemical data such as reaction rate constants and product branching ratios. Applying time-resolved measurement technologies such as time-resolved mass spectrometry based on synchrotron radiation photoionization and time-resolved spectroscopy based on laser absorption (laser setup is under construction), combined with flash photolysis flow reactor, we measure the branch ratios, reaction rate constants, and discriminate the isomers of product for elementary reactions of interest.
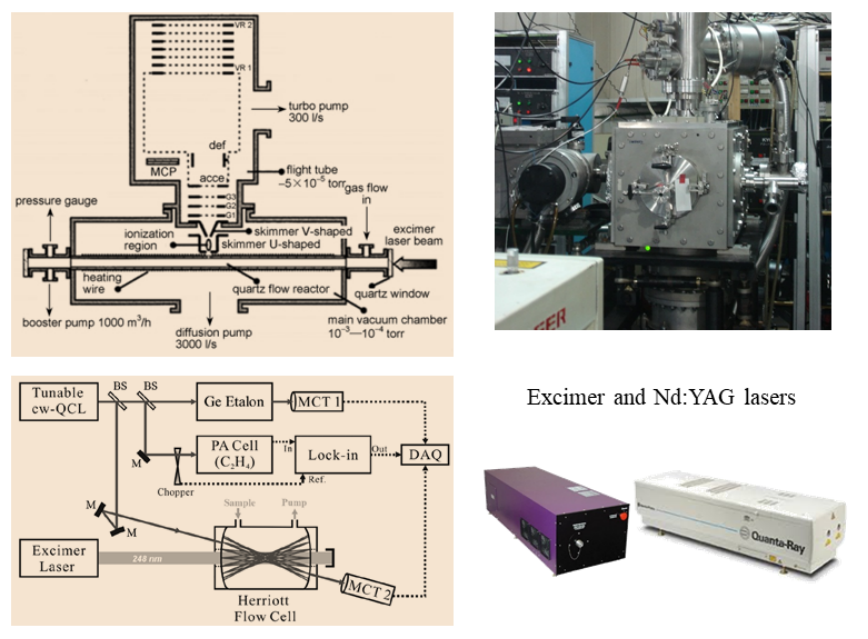
Aerosols are particulate matter suspended in the atmosphere. They can change the abundance and distribution of atmospheric trace gases through heterogeneous chemical reactions and other heterogeneous processes. Organic aerosols make up 20%-90% of the total mass of atmospheric aerosols and significantly affect the global climate and human health. The formation of organic aerosols is mainly caused by the reaction of atmospheric oxidants such as O3 and OH with volatile organic compounds (VOCs). To understand the formation of organic aerosols, especially secondary organic aerosols (SOAs), the chemical composition is one piece of the most important information. For that, we combine the photochemical smog cavity with SR-VUV-PIMS to realize the online analysis of the chemical components of SOAs.
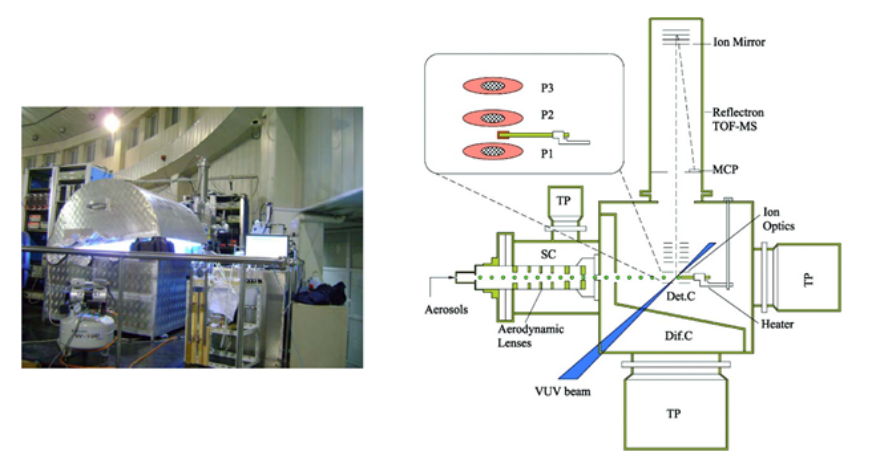
Projects: Atmospheric chemistry, Combustion chemistry, Theoretical calculation and chemical kinetics simulation
Combustion chemistry
■ Gas-phase radical reaction
Exploring the kinetics of gas-phase radical reactions is very important for many interdisciplinary research fields, such as atmospheric chemistry, combustion chemistry, interstellar chemistry, etc. Gas-phase radicals have short lifetime and active chemical properties, making gas-phase free radical reaction a challenging subject. In order to understand the role of related free radicals in the formation of air pollutants (secondary organic aerosols), combustion harmful substances (polycyclic aromatic hydrocarbons, soot particles) and other substances. We use synchrotron radiation-based photoelectron/photoion coincidence (PEPICO) imaging and combine it with homemade flash pyrolysis microreactor to explore important radical elementary reactions in combustion environment.
The reactor used in the experiment is a SiC micro flow tube. The main feature is that the precursor in the reactor can be quickly pyrolyzed. The reactions take place only microseconds in the reactor. The residence time with such order of magnitude is very conducive to retaining free radical intermediates and preventing secondary reactions. The advanced synchrotron radiation photoelectron/photoion coincidence imaging used in the experiment can achieve the targeted differentiation of isomer species with close ionization energies. Equipped with these setups, a number of experimental researches can be carried out, such as alternative fuel single molecule pyrolysis, oxidation reaction, combustion intermediate identification, free radical bimolecular reaction, and catalytic-assisted pyrolysis. The mapping of complex radical reaction network can help us better understand the fuel combustion process and further guide the design of combustion engines, which is of great significance for achieving efficient combustion and clean combustion.
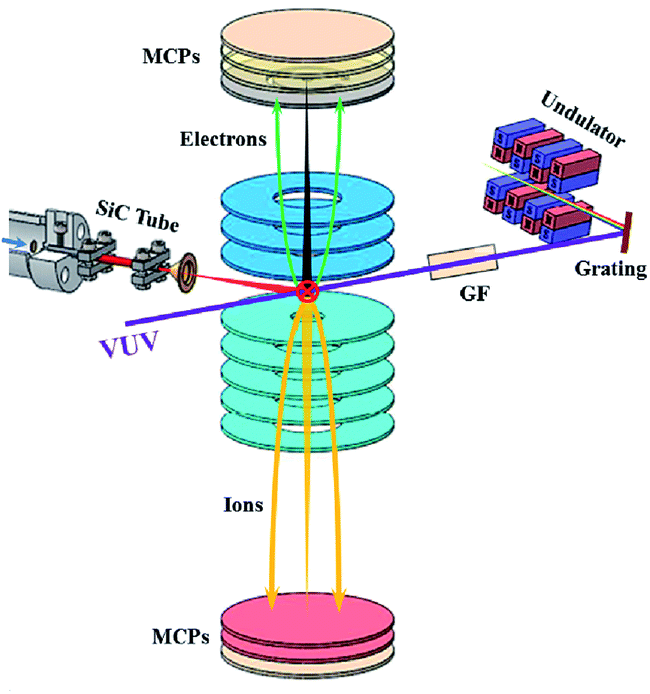
■ Atmospheric-pressure and Low-temperature oxidation combustion
In order to explain the knocking phenomenon of the engine, it is necessary to fundamentally understand the low-temperature oxidative combustion of hydrocarbon fuels. We use jet stirred reactor (JSR) combined with SVUV-PI-MBMS and gas chromatography and other analytical methods to explore the low-temperature oxidation mechanism of hydrocarbon fuels. The experimental measurement of the key species of low-temperature oxidation lays a foundation for inferring the reaction path, establishing the framework mechanism of low-temperature oxidation and developing a kinetic model. Molecular beam sampling technology can realize online analysis of reactive species. The schematic diagram of the SVUV-PI-MBMS experiment is shown in the figure below. The equipment used in the experiment mainly includes a jet stirred reactor made of quartz and the SVUV-PI-MBMS system. There are 4 nozzles with a diameter of 0.3 mm in the middle of the JSR. The fuel/O2/Ar mixture passes through the 4 nozzles to form a uniform jet mixing. On the side of the normal pressure JSR reactor, there is a fused quartz nozzle with a hole diameter of 0.13 mm to sample the reaction product. After sampling, an ultrasonic molecular beam is formed through a nickel colander and reaches the photoionization chamber (II). The neutral molecules are photoionized by synchrotron radiation into ions and then detected by RTOF-MS. The first section of the JSR reactor can also be connected to gas chromatography at the same time for comprehensive analysis of the products.
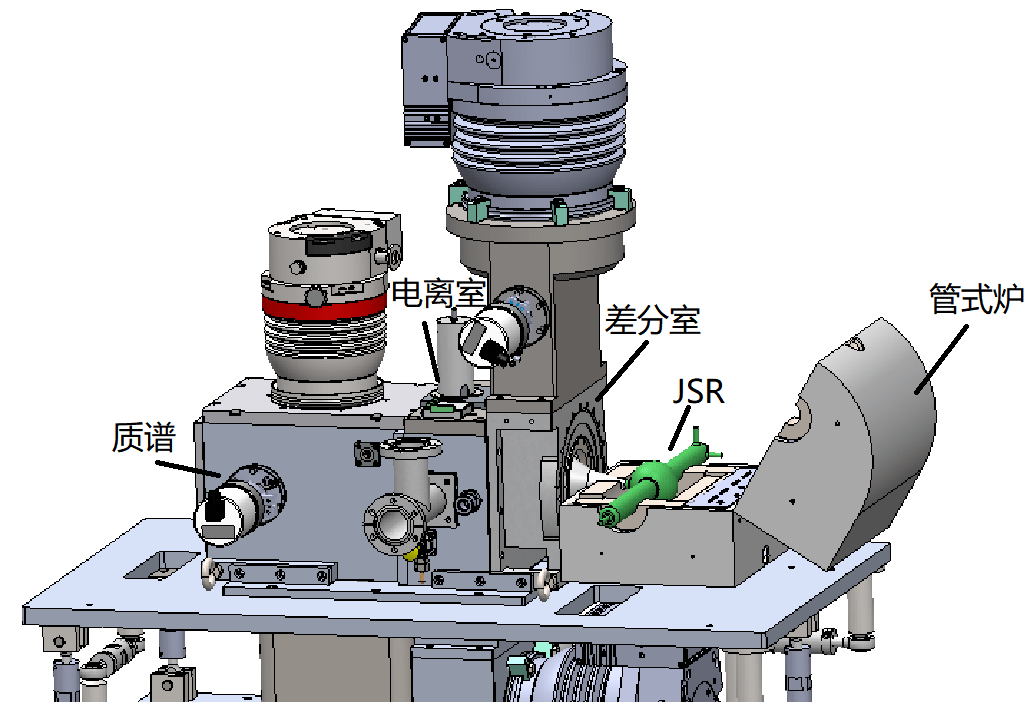
■ High-pressure and low-temperature oxidation combustion
At present, the pressure conditions of the low-temperature oxidation platform based on JSR and SR-VUV-PIMS are around one atmosphere, which is far below the actual pressure conditions in engine combustion. We carry out experimental research on high-pressure and low-temperature oxidation close to the actual engine operating conditions. We have developed a high-pressure JSR that can be combined with SR-VUV-PIMS. The reactor is coupled with a stainless-steel cavity, and the pressure balance is maintained between the inside and outside of the quartz glass reactor is maintained. The metal nozzle sampling and multi-stage differential structure are used to make the transition from high pressure to mass spectrometry vacuum system. The method would be beneficial to explore secondary and tertiary oxygenation under different pressure conditions.
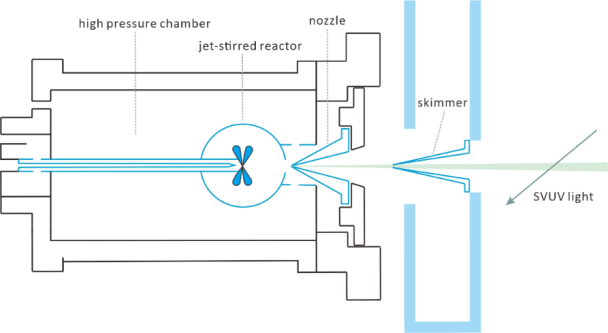
Projects: Atmospheric chemistry, Combustion chemistry, Theoretical calculation and chemical kinetics simulation
Theoretical calculation and chemical kinetics simulation
With the development of computer technology, theoretical calculations are playing an increasingly important role in the research process. It can not only help us understand the progress of the reaction and explain the experimental phenomenon from the reaction mechanism, but also obtain information that is difficult to obtain from experiments such as the reaction branch ratio and the site specificity of the reaction, and can provide important references for experiments and model studies. We use quantum chemical calculations (using software such as Gaussian, Molpro, etc.) and kinetic calculations (using software such as MESS, Polyrate, etc.) to conduct detailed research on the gas-phase reaction mechanism in the atmosphere and combustion process, and build a complete elementary chemical reaction network. The calculations can used to verify the experimental data in a wide range of T-P conditions, build an accurate and reliable atmospheric and combustion reaction kinetic model.
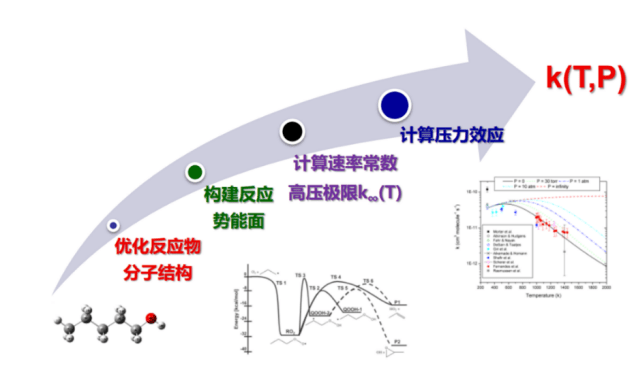
The simulation of combustion reaction kinetics generally requires chemical reaction mechanism, thermodynamic parameters and transport parameters. The reaction mechanism including chemical components, intermediates species and corresponding reaction rates are used to describe the entire chemical reaction network; thermodynamic parameters describing temperature, enthalpy, entropy, heat capacity, etc., are used to calculate equilibrium constants and other information; transportation parameters describing the molecular structure, dipole moment, polarizability, collision diameter, etc. of the species are used to calculate the physical parameters such as the species diffusion constant, thermal conductivity, and molecular viscosity coefficient. With the information above, we can model the target system, solve the energy equation, momentum equation, concentration matrix, etc., and then realize the theoretical description of the gas-phase reaction process. Based on experimental data and theoretical calculation results, we carry out research on the construction method, analysis method, optimization method and simplified method of combustion kinetic model, and construct, develop and verify the combustion kinetic model.
We mainly use gas-phase kinetics simulation software Chemkin, Cantera, OpenSMOKE, etc. As a powerful tool for solving complex chemical reactions, Chemkin has a friendly user interface, fast simulation speed, and a wealth of reactor modules. It is often used for the simulation of combustion processes, catalytic reactions, plasma and other chemical reactions.
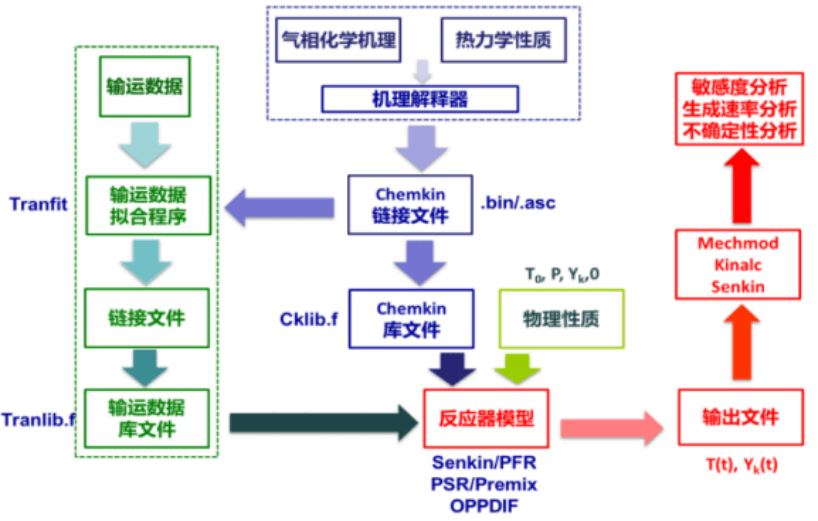
Projects: Atmospheric chemistry, Combustion chemistry, Theoretical calculation and chemical kinetics simulation
Reaction regulation and energy conversion in combustion
Energy and environmental issues are the fundamental issues that significantly affects the development of human society. More than 80% of the world's energy comes from the combustion of fossil fuels, and however the combustion process is also an important source of primary pollutants in the atmosphere. How to understand the combustion process, improve the efficiency of combustion and reduce the emission of pollutants has become core interests for scientists and engineers. In addition, the pollutants emitted into the atmosphere from the combustion process and biological sources are mainly volatile organic compounds. Under atmospheric conditions, they will undergo secondary chemical reactions with radicals to generate ozone and aerosol particles (such as PM2.5). It is the main source of secondary pollutants in the atmosphere.
Combustion reaction regulation is an effective way to achieve high-efficiency combustion and low-pollution emissions. Combustion reaction control has been listed as a priority development area in the "13th Five-Year Plan". Our focus is catalytic assisted combustion and combustion reaction regulation using non-equilibrium plasma.
Projects: Catalytic assisted combustion, Plasma catalysis and energy conversion
Catalytic assisted combustion
Environmental protection and air improvement have become hot areas that attract much attention in recent years. Volatile organic compounds (VOCs) are considered to be the main contributors to air pollution. They not only indirectly act as precursors of ozone or photochemical smog, but also directly cause damage to the environment or the human body. Catalytic combustion is a green and efficient means to convert VOCs into pollution-free CO2 and H2O. The methods of catalyst synthesis and control have become mature, but for the study of the gas-solid interface reactions, the widely used optical spectroscopy methods can only detect and analyze the functional groups of surface species. The detailed catalytic reaction mechanism has always been missing.
Industrial catalytic reactions are mostly carried out under high or normal pressure conditions. Under such conditions, it is difficult to observe free radicals and intermediates in the catalytic process. However, the species are the key to understand the catalytic reaction. We use the tunable synchrotron VUV radiation source combined with homemade atmospheric and low-pressure combustion reactors to explore the catalytic auxiliary combustion process of fuels and realize the catalytic conversion of harmful substances. We have successfully detected formaldehyde, an important intermediate of MTO in the industrial system. Using the high-pressure JSR device combined with SR-VIV-PIMS, we explored the catalytic experiment of synthesis of aromatics from syngas, and successfully detected important oxygen-containing intermediates that cannot be seen in GC-MS. Currently, we are implanting catalyst design on the conversion characterized by diffuse reflectance FTIR spectroscopy and SR-VUV-PIMS. We hope to explore the structure-activity relationship of the gas-solid reaction and address a series of questions like how to achieve the catalytic conversion of CO2 to fuel, and how to achieve the catalytic conversion of VOCs.
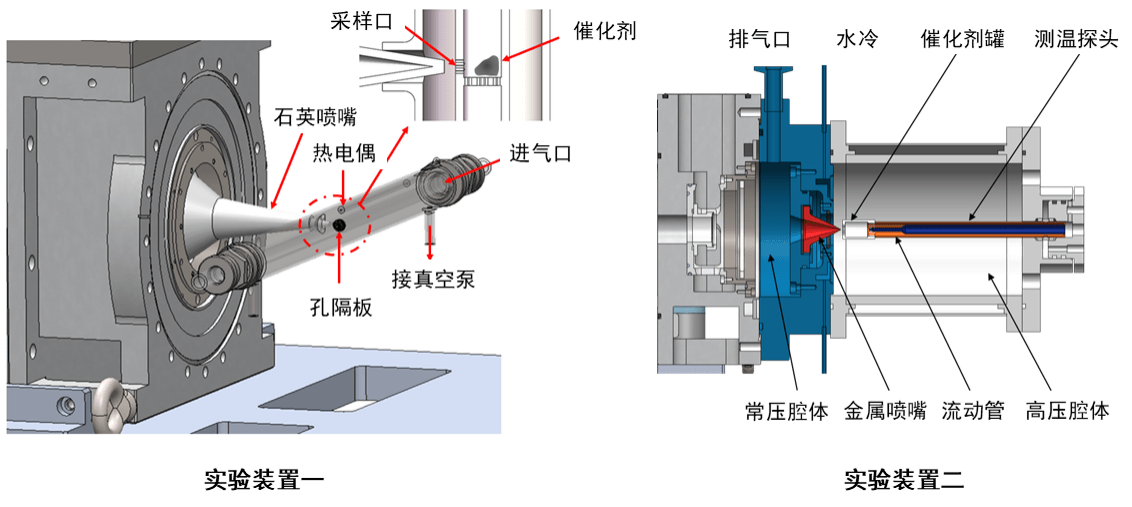
To better understand the mechanism of gas-phase catalytic reaction, we have newly built a research platform for flash pyrolysis catalytic reaction, which is combined with advanced photoelectron photoion coincidence imaging technology. The experimental setup is shown in the figure. This method is a powerful tool for identifying isomers in catalytic assisted combustion.
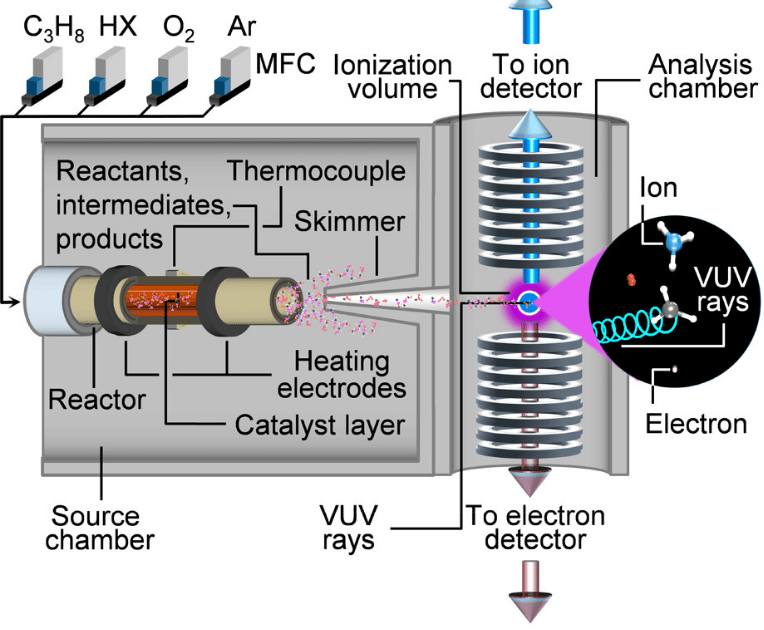
Projects: Catalytic assisted combustion, Plasma catalysis and energy conversion
Plasma catalysis and energy conversion
High-efficiency conversion of low-grade energy into liquid fuels and high-value-added chemicals can fundamentally help the energy conservation and CO2 emission reduction, while achieving the purpose of efficient use of. At present, the thermal catalysis and plasma discharge are main method to convert the low-grade energy materials. However, in the method of thermal catalysis, high temperature is required, and the catalyst is easily deactivated by coking and carbon deposition. In the method of plasma discharge, normal T-P operation conditions can be realized, and the method is relatively cheap, but the product selectivity is low. The plasma discharge can be combined with the catalyst working at room temperature, thereby improving the selectivity of the target product.
Therefore, we use plasma synergistic catalysis technology to efficiently convert low-grade energy materials into liquid fuels and high-value-added chemicals. While improving combustion efficiency, we reduce pollutant emissions, so as to achieve the purpose of energy saving and emission reduction. At the same time, with the help of synchrotron radiation photoelectron spectroscopy, synchrotron radiation X-ray imaging, synchrotron radiation infrared and other technologies, the mechanism of low-grade energy plasma catalytic conversion is studied, so as to propose a further mechanism of plasma co-catalysis for low-grade energy conversion.
The experimental system is mainly divided into six parts, namely, gas supply system Ⅰ and Ⅱ, dielectric barrier discharge cooperative catalytic reaction system Ⅲ, energy supply system Ⅳ, product analysis system Ⅴ and combustion detection system Ⅵ.
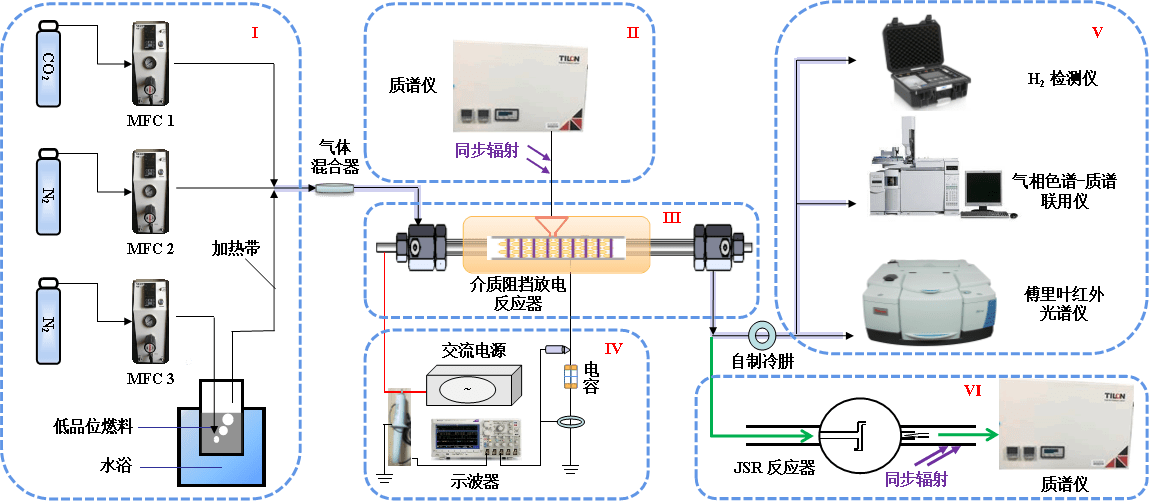
Projects: Catalytic assisted combustion, Plasma catalysis and energy conversion
Component analysis of complex chemical systems
For ordinary samples containing dozens or hundreds of components, chromatography and conventional mass spectrometry (such as time-of-flight mass spectrometry) are usually sufficient for separation and detection. However, in the fields of combustion, biomass, atmospheric environment, etc., the sample may contain thousands of organic molecules, the relative molecular mass can reach thousands, and the elements contained are not limited to C, H, O, and may contain P, S , Cl, F and other elements. The complex substance types and element composition result in dozens of substances in a unit mass. The analysis of this type of sample places extremely high requirements on the resolving power of the mass spectrometer.
The Fourier Transform Ion Cyclotron Resonance Mass Spectrometer (FT-ICR-MS) is a mass spectrometry instrument that measures the mass-to-charge ratio of ions according to the ion cyclotron resonance frequency in a given magnetic field. The Bruker solariX FT-ICR-MS, located in the State Key Laboratory of Fire Science, has a 7T strong magnetic field and is equipped with three ion sources: ESI, APCI, and APPI. It can "soft" ionize samples and obtain clean spectra with almost no debris. Soft ionization combined with ultra-high mass resolution helps to realize the analysis of different kinds of complex organic matter components, and the computer automatically analyzes the spectrum to give the molecular formula of the measured substance. Application areas include combustion and flame retardant, environmental science, atmosphere, petroleum (bio-oil), etc. The specific parameters are as follows:
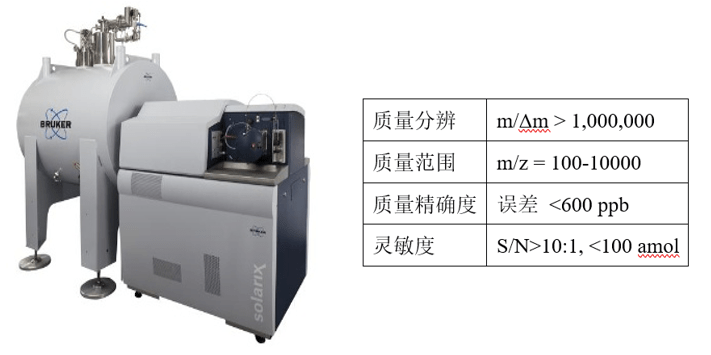
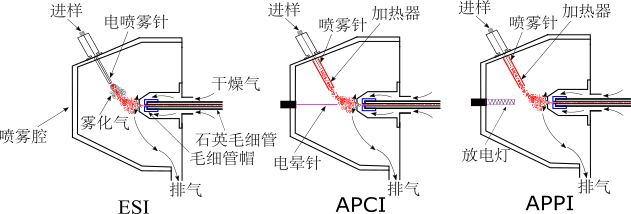